“`html
The Environmental Footprint of Electric cars: A Comprehensive Analysis
The Environmental Footprint of Electric Cars: A Comprehensive Analysis
Electric vehicles (EVs) have surged in popularity, touted as a key solution to mitigating climate change and reducing air pollution. However, the environmental impact of EVs is a complex issue, encompassing the entire lifecycle from raw material extraction to end-of-life disposal. This article delves into the various facets of EV environmental impact, providing a comprehensive analysis of their benefits and drawbacks.
1. Greenhouse Gas Emissions: Well-to-Wheel Analysis
One of the primary arguments for EVs is their potential to reduce greenhouse gas (GHG) emissions. To accurately assess this, we must consider a “well-to-wheel” analysis, which encompasses emissions from:
1.1. Upstream Emissions: Material Extraction and Manufacturing
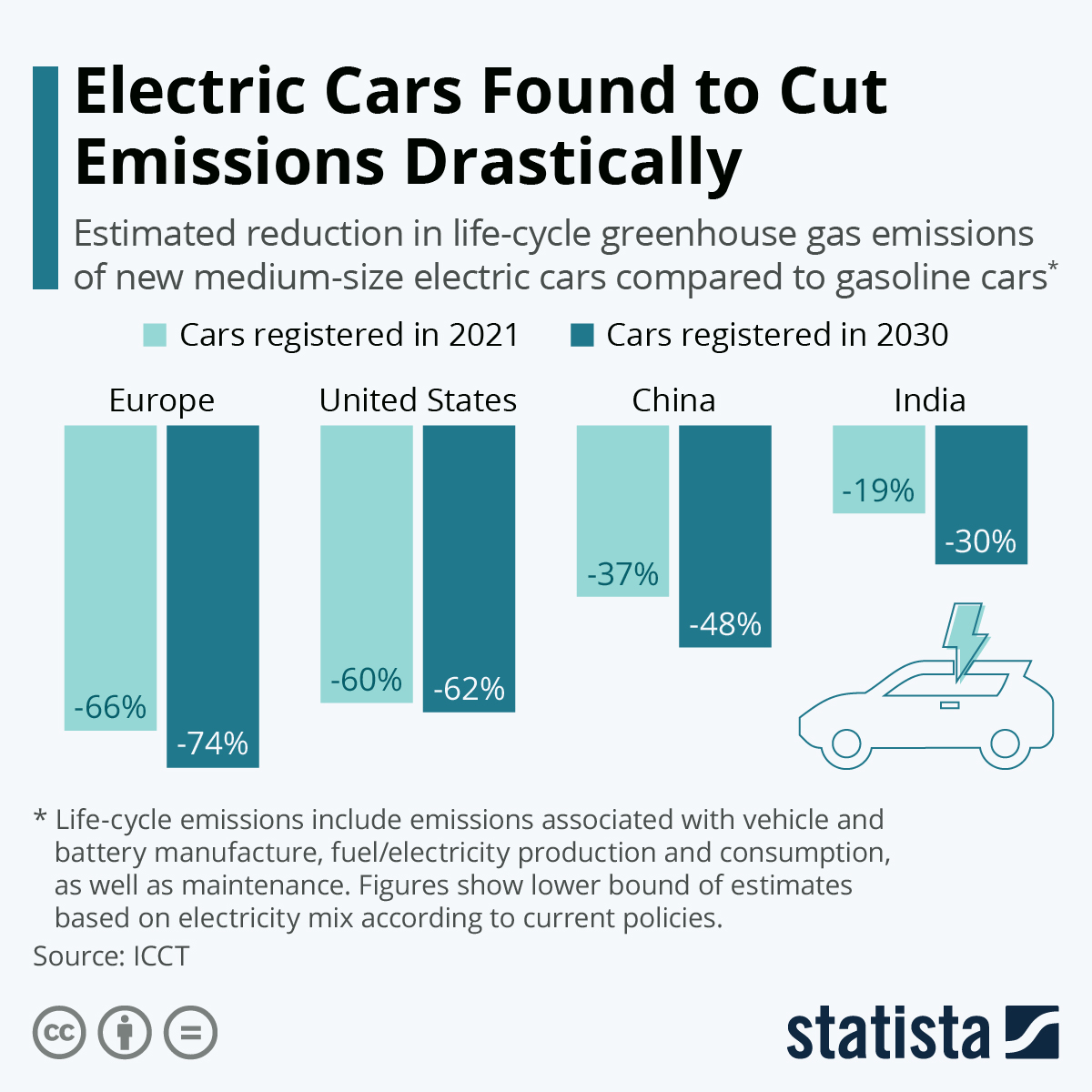
The production of EV batteries and components involves significant energy consumption and emissions. Mining for lithium, cobalt, nickel, and other critical minerals can lead to habitat destruction, water pollution, and substantial CO2 emissions. The manufacturing process itself, including battery cell production and vehicle assembly, also contributes to GHG emissions. The location of these manufacturing facilities and their energy sources are critical factors.
1.2. Operational Emissions: Electricity Generation and Consumption
The operational emissions of an EV depend heavily on the electricity grid’s carbon intensity. In regions with a high proportion of renewable energy sources (solar, wind, hydro), EVs can achieve significantly lower operational emissions compared to internal combustion engine vehicles (ICEVs). Conversely, in areas reliant on coal-fired power plants, the benefits may be diminished or even negated. The efficiency of the EV drivetrain and the driving style also influence energy consumption.
1.3. Downstream Emissions: End-of-Life and Recycling
The end-of-life management of EV batteries is a growing concern. Proper recycling is crucial to recovering valuable materials and preventing environmental contamination. However, current recycling infrastructure is limited, and the process can be energy-intensive. The disposal of other vehicle components also contributes to the overall environmental footprint.
2. Air Quality and Public Health
EVs offer substantial improvements in local air quality, particularly in urban areas. They eliminate tailpipe emissions of pollutants such as nitrogen oxides (NOx), particulate matter (PM), and volatile organic compounds (VOCs), which are major contributors to respiratory diseases and other health problems.
2.1. Urban Air Pollution Reduction
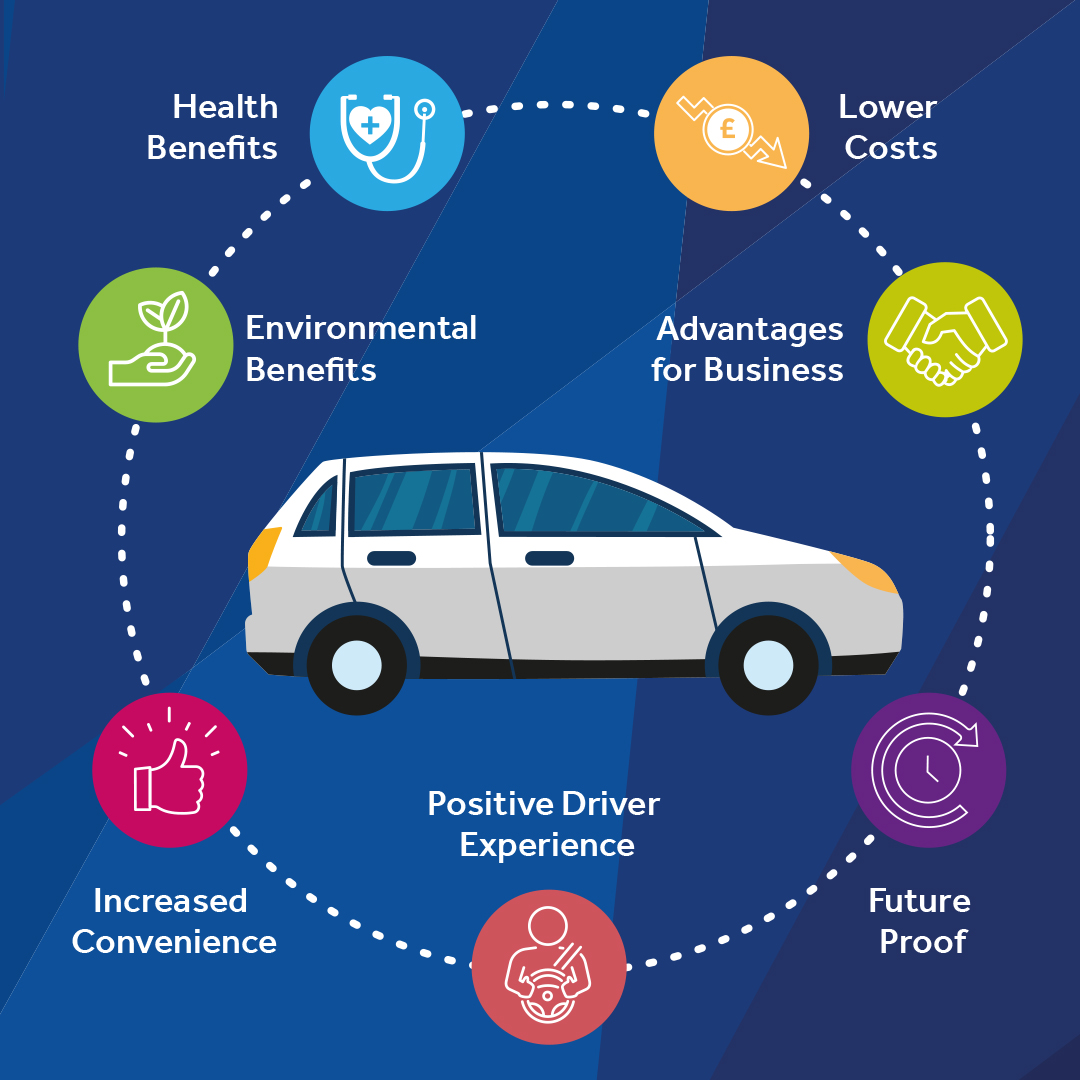
The shift to EVs can significantly reduce urban air pollution, leading to improved public health outcomes. This is particularly important in densely populated areas where traffic congestion and air pollution are prevalent. The reduction of NOx and PM emissions can alleviate respiratory problems and reduce the incidence of cardiovascular diseases.
2.2. Indirect Air Pollution: Power Plant Emissions
While EVs eliminate tailpipe emissions, they may shift air pollution to power plants. The impact on air quality depends on the power plant’s location and emission controls. However, centralized power plants often have better emission controls than individual vehicles, and the shift towards renewable energy sources further reduces this indirect pollution.
3. Resource Consumption and Sustainability
The production of EVs requires significant resources, raising concerns about sustainability. The demand for critical minerals like lithium, cobalt, and nickel is increasing rapidly, leading to potential supply chain vulnerabilities and environmental impacts.
3.1. Critical Mineral Extraction and Impacts
Mining for critical minerals can lead to deforestation, soil erosion, water pollution, and habitat destruction. The social impacts of mining, including human rights abuses and labor exploitation, are also a concern. Sustainable sourcing and responsible mining practices are essential to mitigating these impacts.
3.2. Battery Manufacturing and Material Intensity
EV batteries are material-intensive, requiring large quantities of lithium, cobalt, nickel, and other materials. The environmental impact of battery manufacturing can be reduced through the use of recycled materials, improved battery chemistry, and more efficient production processes. Research and development efforts are focused on developing batteries with lower material intensity and higher energy density.
3.3. Water Consumption and Pollution
The production of EVs, particularly battery manufacturing and mineral extraction, can consume significant amounts of water and contribute to water pollution. Lithium extraction, for example, can deplete local water resources and contaminate groundwater. Sustainable water management practices are crucial to minimizing the environmental impact of EV production.
4. Land Use and Ecosystem Impacts
The environmental footprint of EVs extends beyond emissions and resource consumption to include land use and ecosystem impacts.
4.1. Mining and Habitat Destruction
Mining operations can lead to habitat destruction, deforestation, and biodiversity loss. The expansion of mining activities to meet the growing demand for EV batteries can have significant ecological consequences. Responsible mining practices and the protection of sensitive ecosystems are essential.
4.2. Renewable Energy Infrastructure
The transition to EVs requires a significant expansion of renewable energy infrastructure, including solar farms, wind turbines, and hydropower plants. While renewable energy sources have lower GHG emissions than fossil fuels, they can also have land use and ecosystem impacts. Careful planning and siting are crucial to minimizing these impacts.
5. Lifecycle Assessment and Circular Economy
A comprehensive lifecycle assessment (LCA) is essential to evaluate the overall environmental impact of EVs. This involves analyzing the environmental impacts at each stage of the vehicle’s lifecycle, from raw material extraction to end-of-life disposal. A circular economy approach, focused on reducing, reusing, and recycling materials, is crucial to minimizing the environmental footprint of EVs.
5.1. Importance of Lifecycle Assessment
LCA provides a holistic view of the environmental impacts of EVs, allowing for informed decision-making and the identification of areas for improvement. It helps to avoid shifting environmental burdens from one stage of the lifecycle to another. Standardized LCA methodologies are needed to ensure consistent and comparable results.
5.2. Battery Recycling and Second-Life Applications
Battery recycling is crucial to recovering valuable materials and reducing the environmental impact of EV batteries. Second-life applications, such as using retired EV batteries for stationary energy storage, can extend their useful life and reduce waste. Developing efficient and cost-effective recycling technologies is essential.
5.3. Sustainable Supply Chains and Material Innovation
Building sustainable supply chains for EV components and materials is essential to minimizing environmental and social impacts. This includes responsible sourcing, transparency, and traceability. Material innovation, such as developing batteries with lower material intensity and using alternative materials, can also contribute to sustainability.
6. Policy and Technological Advancements
The environmental impact of EVs can be further reduced through policy interventions and technological advancements. Government policies, such as subsidies, regulations, and infrastructure investments, can accelerate the transition to EVs and promote sustainable practices. Technological advancements, such as improved battery technology, more efficient manufacturing processes, and smart charging infrastructure, can also contribute to reducing the environmental footprint of EVs.
6.1. Government Policies and Incentives
Government policies play a crucial role in promoting the adoption of EVs and supporting the development of sustainable infrastructure. This includes subsidies for EV purchases, investments in charging infrastructure, and regulations to phase out ICEVs. Policies that promote the use of renewable energy sources for electricity generation are also essential.
6.2. Technological Innovations in Battery Technology
Advances in battery technology, such as solid-state batteries, lithium-sulfur batteries, and sodium-ion batteries, can improve energy density, reduce material intensity, and enhance safety. Research and development efforts are focused on developing batteries that are more sustainable, affordable, and efficient.
6.3. Smart Charging and Grid Integration
Smart charging technologies can optimize EV charging to minimize grid impacts and maximize the use of renewable energy. This includes time-of-use pricing, vehicle-to-grid (V2G) technology, and demand response programs. Smart charging can also help to integrate EVs into the electricity grid and support the transition to a more sustainable energy system.
7. Conclusion: Balancing Benefits and Challenges
Electric vehicles offer significant potential for reducing greenhouse gas emissions and improving air quality. However, their environmental impact is complex and multifaceted. Addressing the challenges associated with material extraction, manufacturing, and end-of-life management is crucial to realizing the full environmental benefits of EVs. A holistic approach that considers the entire lifecycle of EVs, promotes sustainable practices, and fosters technological innovation is essential for a truly sustainable transportation future.
“`